Link zur deutschen Version: Verhalten von statisch unbestimmten Stahlbetontragwerken im Brandfall
Large deformations are often observed in experimental fire tests on statically determinate structures, which suggests a large deformation capacity. Deformations are triggered initially by the temperature gradient between the surface exposed and the surface unexposed to fire, and subsequently increased by the temperature-dependent degradation of material properties. However, in statically indeterminate structures, rotations and/or axial expansions are restrained, e.g. at intermediate supports of continuous slabs or beams or at fixed end supports. Due to these restraints, temperature differences within the cross-section cause indirect actions whose magnitude depends on the static system, the stiffness of its members and the cross-sectional resistance.
The main structural effects of fire are highlighted in Figure 1, which shows the consequences of a fire exposure at the bottom of a statically indeterminate two-span slab strip documented by Kordina and Wesche (1979). Figure 1a shows the elastic bending moment distribution along the slab axis (i) at ambient temperature (denominated Md,el), assuming a uniformly distributed load and a ratio of design load at ambient temperature to that under fire conditions of qd/qd,fi = 1.4, and (ii) under fire conditions (denominated Mfi) at different fire exposure times t = [0, 5, 10, 15, 30] min, taking into account the actual load introduction points during the test. Kordina and Wesche (1979) state that the slab strip was designed with an intended moment redistribution of 15% of the maximum elastic hogging moment at ambient temperature; the redistributed bending moment distribution is indicated with the dashed line (denominated Md,pl) in Figure 1a. Figure 1b shows the variations of the bending moment and the support force at the intermediate support during exposure time t, indicating that positive curvatures caused by the fire exposure at the bottom side of the slab lead to a drastic increase of the hogging moment and vertical reactions at the intermediate support already at short exposure time. Figure 1c shows the measured maximum deflection in the span and an estimation of the corresponding plastic rotation at the intermediate support (calculated based on the deflections in the span) during exposure time t. Obviously, as soon as the yield moment was reached after 15 min fire exposure, significant plastic rotations took place, amounting to almost 120 mrad after 90 min, which by far exceeded those acceptable at ambient temperature (cf. provisions in the EN 1992-1-1).
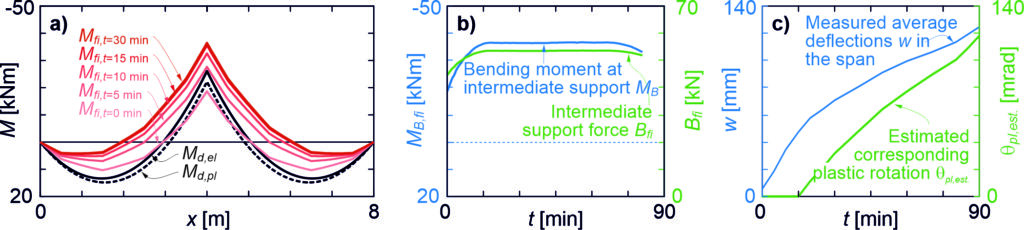
Within the framework of revising the EN 1992-1-2 on structural fire design, we reviewed several experimental campaigns on statically indeterminate members. We mainly focused the review on experimental campaigns led by Professor Karl Kordina and carried out at TU Braunschweig almost 50 years ago: Ehm et al. (1970) and Wesche (1974) reported several fire tests on continuous beams, while Kordina and Wesche reported nine fire tests on two-span slab strips. Figure 2 shows Beams 1 and 4 after their testing carried out by Wesche and Figure 3 shows Slabs 1,2, 4, 5 and 9 after their testing carried out by Kordina and Wesche.
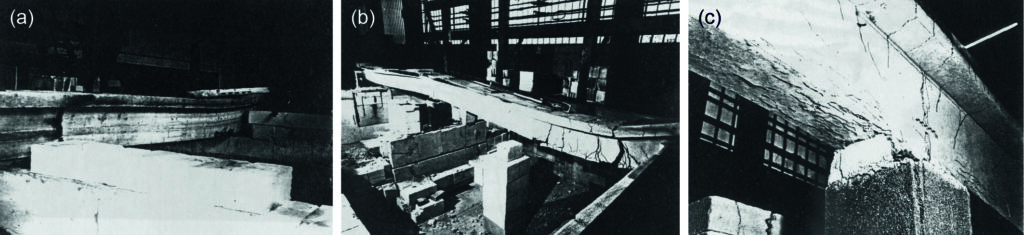
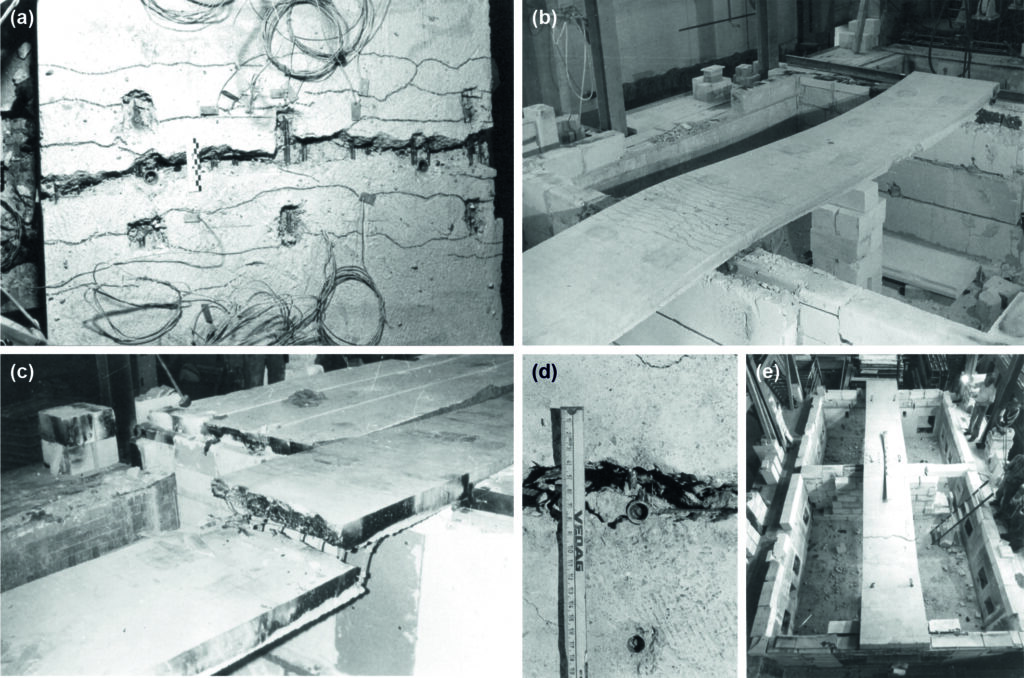
The findings from these experiments at TU Braunschweig had a direct impact on the design and detailing rules for statically indeterminate reinforced concrete beams and slabs given in the EN 1992-1-2:
- The hogging moment reinforcement needs to be extended into the span of continuous beams and slabs, as shown in Figure 4. In four experiments of Ehm et al. and four experiments of Kordina and Wesche, cracking at the end(s) of the top reinforcement in the region of the intermediate support occurred early in the experiment, even before the reinforcement yielded at the intermediate support (see Figure 3c). Such a failure may occur if the differences in the bending moment curves between ambient temperature and in fire conditions (compare also Figure 1a) are ignored when detailing the reinforcement.
- When considering this detailing rule of extended hogging moment reinforcement, static indeterminacy is generally advantageous for beams, allowing for reduced axis distances compared to static determinacy.
- The tabulated design data of EN 1992-1-2 for statically indeterminate members may be used up to a moment redistribution of 15% from the intermediate support to the span in ambient temperature design. EN 1992-1-2 indirectly limits overly low reinforcement ratios at the intermediate support by limiting the redistribution.
- A minimum reinforcement ratio of 0.5% is required over intermediate supports in continuous slabs where limited rotation capacity (cold worked reinforcement) or increased rotation demand (one-way continuous slab) is expected, and
- Two-way slabs and axially restrained slabs generally incorporate sufficient redundancy to avoid a bending failure under fire conditions, as Wesche (1985) and Wiese (1987) pointed out in their dissertations. For flat slabs, EN 1992-1-2 asks for (i) an increased thickness (e.g. hs = 200 mm for R90) than for slabs with line support and (ii), for R90 and higher, a minimum percentage of 20% of the top reinforcement in the column strip to be continuous over the entire span.
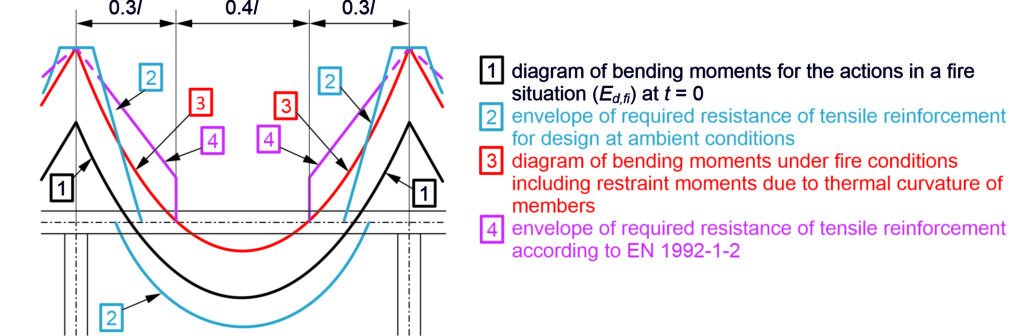
While respecting these design and detailing rules, continuous beams with reduced axis distances than for simply supported beams may be considered safe according to the current EN 1992-1-2 and sufficiently validated by experimental results. However, the design provisions for continuous slabs contain a considerable amount of extrapolation and engineering judgement, given the rather scarce evidence for one-way continuous slabs: only three of nine continuous slab strips of Kordina and Wesche survived longer than expected when using tabulated design data given in EN 1992-1-2. Accordingly, the rotation capacity of continuous slabs may not be taken for granted if no axial restraint can be mobilised (e.g. for typical two-span tunnel ceilings) nor two-way load carrying conditions prevail (many precast solutions).
Furthermore, plastic hinges caused by the high restraint moments under fire conditions are relevant for reliably modelling the global response of concrete structures under (partial) fire exposure. Therefore, we developed a model to assess the behaviour of members subjected to bending under fire conditions. The model builds on the Tension Chord Model, which was developed by Sigrist (1995) for studying the rotation capacity of members subjected to bending at ambient temperature. Our model is based on constitutive material properties given by EN 1992-1-2 and some complementary considerations concerning the biaxial compressive strength of concrete and strain hardening of reinforcement. The structural system is analysed using cross-sectional moment-curvature relationships established with the constitutive material properties and ensuring global equilibrium and compatibility.
Figure 5 compares (a) the bending moments MB at the intermediate support, (b) the maximum deflections wSpan in the span and (c) the reinforcement strains εsm,B averaged over 100 mm at the intermediate support in the slabs tested by Kordina and Wesche. Generally, the model predictions correlate well with the experimental results regarding the fire resistance, the evolution of the bending moments at the intermediate support, as well the deflections in the span. The comparison between test results and model predictions reveals a considerable effect of the concrete aggregate used mainly caused by the corresponding concrete thermal expansion.
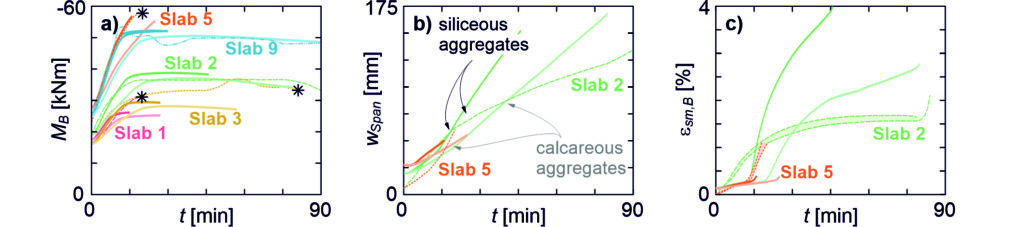
With the developed model, we conducted a parametric study aiming to (i) identify the most influential parameters for the structural fire behaviour of statically indeterminate systems and (ii) discuss whether design rules provided by EN 1992-1-2 can be safely applied to slabs and beams whose dimensions differ significantly from those featured in the tests used for validation. The parametric study explored the effect of uncertainties related to the modelling of statically indeterminate systems because not only the stiffness and strength, but also the internal actions are highly influenced by simplified material models. The following parameters are identified as the most influential ones:
- ductility of reinforcing steel (higher rotation capacity with higher ductility class),
- reinforcement ratio (higher rotation capacity with higher reinforcement ratio),
- concrete strength (higher rotation capacity with lower strength) and aggregate type of concrete (lower rotation demand with calcareous aggregates than with siliceous aggregates) and
- axial and rotational end restraint conditions (beneficial for fire resistance).
While engineers can specify the reinforcement ductility, reinforcement ratio and concrete strength in design, the concrete aggregate type generally cannot be specified and the restraint conditions are challenging to quantify. Accordingly, design recommendations given in EN 1992-1-2 and mainly developed at TU Braunschweig under the lead of Professor Kordina focus on the properties that can be influenced by the designer.
Based on our parametric study, the detailing rules given in EN 1992-1-2 are considered to remain valid for practical applications. The rotation capacity is sufficient for most studied slabs with a reinforcement ratio of 0.5% or higher. The detailing rules may not cover all eventualities such as the reduced rotation capacity when using high strength concrete, or large redistributions of shear forces towards the intermediate support. However, they are overall reasonably safe and easily applicable to cover most continuous slabs of current practice.
We also showed in our parametric study that results for modelled statically indeterminate systems are subject to considerable uncertainties if (i) information on the used material is incomplete or (ii) the used models do not or insufficiently cover all necessary information (e.g. lack of providing a hardening branch in the stress-strain relationship for reinforcing steel). Indeed, the concrete aggregate type with its corresponding thermal expansion or the consideration of strain hardening properties and tension stiffening may affect the fire rating across several standard fire resistances when modelling the behaviour of statically indeterminate bending members exposed to fire.
Patrick Bischof
Comment this post on LinkedIn or Instagram