Link zur deutschen Version: Zum Brandingenieurwesen im Stahlbetonbau
A room can be heated by fire to over 1000°C in a few minutes, depending on its size, ventilation conditions and the actual fire load. Increased temperatures induced by fire action cause for reinforced concrete structures:
- heating of the cross-section depending on its thermal material properties,
- degradation of the mechanical material properties (strength and stiffness) depending on the corresponding temperatures in the cross-section,
- an increased propensity to explosive spalling in some cases, and
- altered internal actions and reactions for statically indeterminate structural systems.
Various parameters influence the behaviour of reinforced concrete structures under fire exposure, mainly the fire load, the composition of the concrete and its moisture content, stresses and strains of static or thermal origin, the cross-sectional geometry, as well as the structural detailing. For example, columns are subject to increased deflections or statically indeterminate bending members experience increased shear or support forces depending on the fire exposure, the used material, and the structure. Increased column head deflections were the main reason for the failure of a warehouse in Ghent (1974) (see image), while force redistributions to intermediate columns were partly responsible for the failure of an underground car park in Gretzenbach (2004) (see image).
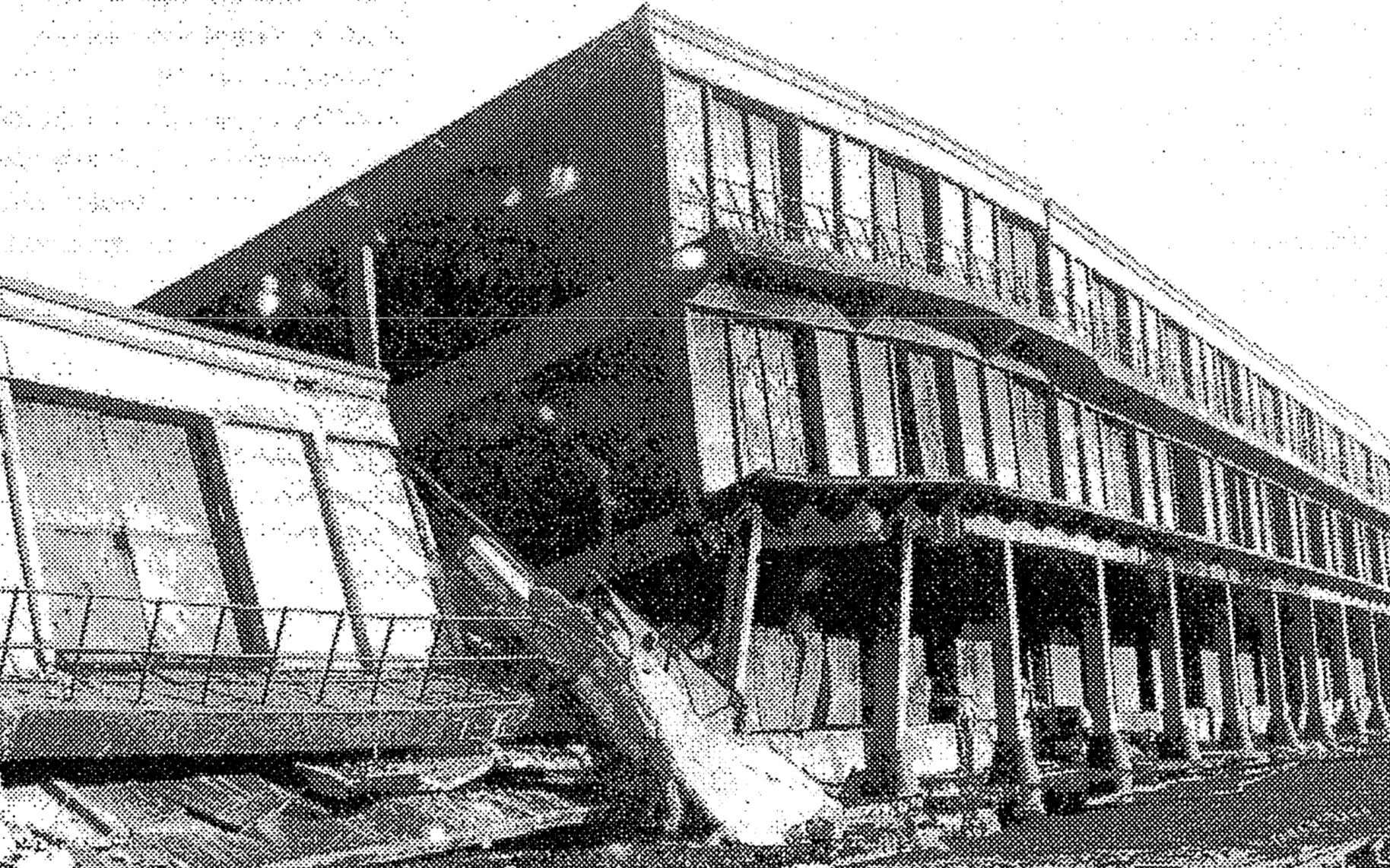
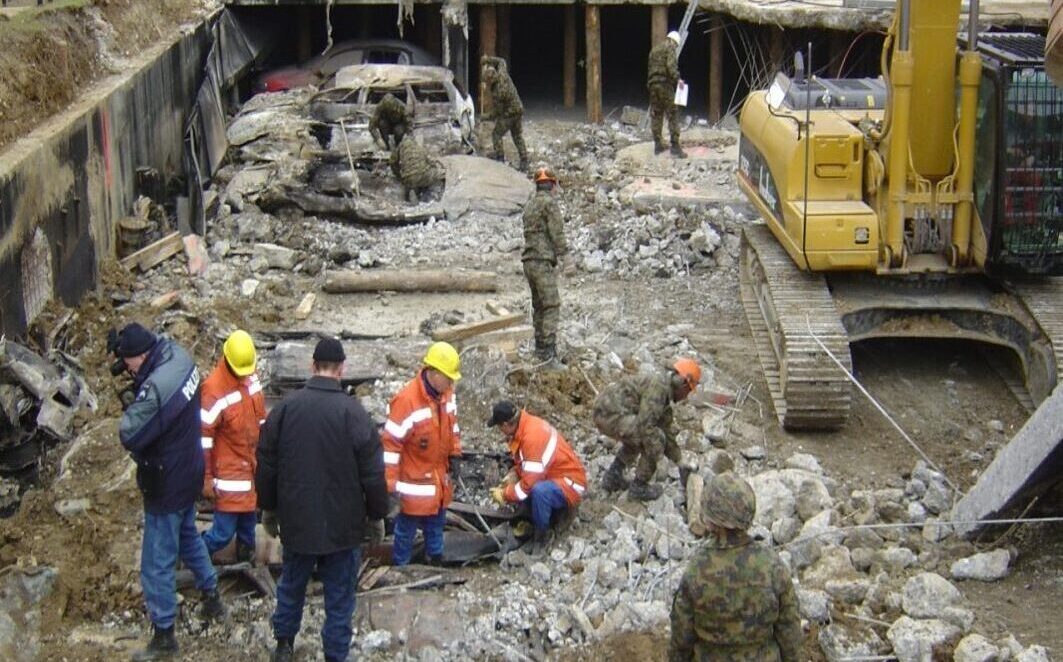
It is often difficult or impossible to explain all phenomena occurring in practical cases of damage caused by fire and in large-scale (representative) fire tests. After extinguishing a fire in buildings, it is only possible to determine which damage occurred at what instant with great uncertainty. In fire tests, only the temperature, deformations, and external forces can reliably be measured to discuss the tested member’s local and global behaviour. Nevertheless, thanks to extensive experimental studies (supplemented by theoretical considerations) on the fire behaviour of reinforced concrete structures between 1960 and 1990 (e.g. at the Technical University of Braunschweig under the direction of Prof. Kordina), it was possible to derive simple tabulated design data, detailing rules, and easily applicable material models to verify the fire resistance under standard fire conditions. Thereby, fulfilling fire safety requirements for reinforced concrete structures is, in most cases, a straightforward engineering task – justifiably because reinforced concrete structures exhibit a favourable behaviour in case of fire with the cross-sectional dimensions and concrete covers currently adopted. The favourable behaviour of reinforced concrete in case of fire is mainly because (i) concrete heats up comparatively slowly and consequently protects the reinforcement from being heated, (ii) reinforced concrete cross-sections are massive compared to cross-sections made of other building materials and (iii) concrete does not burn and, consequently, does not contribute to the fire load. Accordingly, the fire resistance of concrete structures is guaranteed in most cases by conceptual decisions and quick verifications using tabulated design data.
An excellent example of this favourable behaviour is the fire of the seven-storey car park of the Liverpool Echo Arena (dimensions: 70 m x 60 m) in 2017. The fire and fire load in Liverpool was unprecedented in its extent (up to 1400 vehicles burnt out during several hours) because the initial fire in one car quickly spread to other vehicles. The car park consisted of columns, beams, staircase cores, and prefabricated ribbed floor elements. Some of the ribbed floor elements were severely damaged or collapsed completely, but the rest of the structure remained practically undamaged (see image, reference: Structural-Safety).
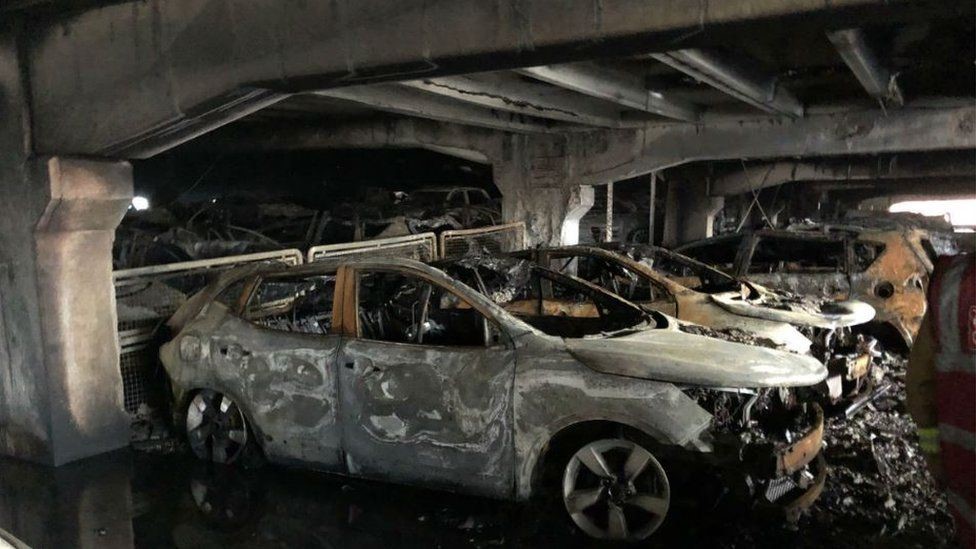
However, simple tabulated design data may be insufficient or inapplicable for designing or assessing structures with a high risk of damage or personal injury (e.g. hospitals, high rise buildings, tunnels) and members with high slenderness or of new concrete types. In such cases, an in-depth understanding of the fire behaviour of reinforced concrete buildings is necessary to conscientiously carry out the design or assessment without tabulated design data. Keeping the efforts to achieve carbon neutrality in mind, studies going beyond the application of tables may be increasingly necessary because:
- the use of alternative engines such as lithium-ion battery engines generate fire curves whose consequences on reinforced concrete structures have not yet been widely studied (NB: today’s tabulated design data of EN 1992-1-2 and SIA 262 only apply for the standard fire curve),
- new ways will be (must be) found to efficiently produce members and structures with less massive reinforced concrete cross-sections than today – such as with filigree ribbed structures –, and
- newly developed types of concrete (such as recycled aggregate concrete, dense concretes, (ultra)high-strength concretes, etc.) with higher propensity to explosive spalling will be (must be) used increasingly.
Explosive spalling of mainly precast concrete under fire conditions has brought fire safety engineering to the table of the civil engineer in Switzerland over the last ten years – and also to my table when starting my scientific work in Prof Walter Kaufmann’s group at ETH Zurich. After initial work on this issue with the idea of expanding the basis for the Corrigenda C1 of SIA 262 in 2017, we could expand our knowledge on fire safety engineering continuously over the last four years while working in the project team to revise the current EN 1992-1-2.
Keeping the relevance of fire safety engineering in mind, fire safety engineering is integrated into the course “Advanced Structural Concrete” (Master’s programme in Civil Engineering at ETH Zurich) and, since 2021, also into the MAS studies on Fire Safety Engineering (established and organised by the Chair of Timber Structures from ETH Zurich). We introduce the material and structural behaviour under fire conditions and present the application, strengths, and limitations of the verification methods available in the standards. Based on this knowledge, we foster the ability to check the plausibility of the results obtained by advanced design methods. Furthermore, we give an overview for the evaluation of the fire safety assessment of existing structures. Last but not least, we want to convey an understanding of the assumptions underlying the standard to make future designers recognise the engineering challenges not covered by them.
Patrick Bischof
Comment this post on LinkedIn or Instagram