The title of this blog entry is the most condensed possible description of what has kept me busy the past five years. Last December I successfully defended my thesis on this topic, which I completed under the supervision of Prof. Dr. W. Kaufmann. In this post, I will try to give you a slightly less condensed version of the thesis’ content, by providing answers to the questions that follow.
What is the problem and why is it relevant?
The reason for which in 2021 we are still discussing a load case for which code provisions exist, is linked to the fact that developed countries today are being faced with the challenge of ageing infrastructure. With traffic intensity as well as traffic loads having increased since their construction, many infrastructure objects need to be re-assessed in order to ensure their structural safety. Current design codes and guidelines are based on past research and associated models that were conceived with the purpose of safely designing new structures. They are therefore typically characterised by a certain degree of conservativism. While a certain degree of conservatism is certainly appropriate when designing new structures, as it comes along with an increase of robustness and redundancy at little extra cost, this does not apply to the safety of existing structures.
One of the load cases these structures have to be assessed for, as the title suggests, is the combination of in-plane shear and transverse bending. It is clear that loading a bridge box girder causes longitudinal bending and therefore longitudinal in-plane shear. At the same time, the loads applied to the deck also have to be transferred in the transverse direction causing bending in the section. As the web is monolithically connected to the deck it receives a large portion of the bending moment, which it is required to transfer in addition to the in-plane shear arising from the longitudinal load transfer.

What are the most widely used models and why was additional research required?
A group of approaches, which can be classified as rigid-ideally plastic models, make use of the observation that a potential bending about the vertical axis and or twisting deformation of the web element cannot occur as it is restrained by the deck, bottom flange and adjacent web elements, as well as by the presence of support and/or intermediate diaphragms. These deformations are therefore restrained and instead generalised reactions arise which take any value such as to ensure compatibility with these kinematic restraints.

The fact that the twisting moment can take arbitrary values, enables the lateral shift of the compression field, which is responsible for the transfer of in-plane shear towards the flexural compression side. This allows the stirrups on the flexural tension side to be utilised almost entirely for the transfer of bending moment, leading to considerably greater capacities.
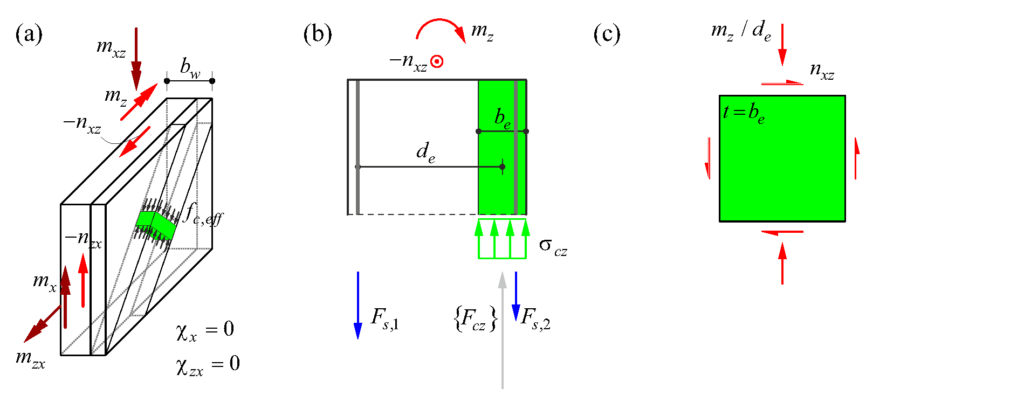
These rigid-ideally plastic models have the potential to provide good estimates of the web’s load bearing capacity. However, their applicability on members with very low stirrup reinforcement ratios is limited, since their fundamental assumption of sufficient deformation capacity cannot, in those cases, be deemed satisfied without an explicit verification.
Furthermore, these models have been validated on a very limited amount of experimental data. As a result, their second fundamental assumption of a lateral shift of the compression field, enabled by the kinematic restraints the web is subjected to, was never verified experimentally.
Another aspect that deserves attention is the fact that the transverse bending moments in the web are not known beforehand, but rather depend on the stiffness of the web relative to the deck. This effect has also not been investigated so far at all, neither theoretically nor experimentally. If the web exhibits sufficient deformation capacity, i.e. does not fail prematurely in a brittle manner but gradually loses its bending stiffness, transverse bending moments caused by eccentric traffic loads could be transferred from the web to the bridge deck, and transverse bending moments due to the distortion of the cross-section could be reduced as well (at the expense of an increase in warping torsion).
How were the knowledge gaps filled?
The knowledge gaps were filled by performing large-scale tests on panels that represented isolated web elements of a box-girder bridge in the Large Universal Shell Element Tester.
The Large Universal Shell Element Tester is a testing facility inspired by two testing facilities at the University of Toronto, the capabilities of which it combines in order to enable the performance of large-scale tests on reinforced concrete shell elements with dimensions of 2 m x 2 m and a variable thickness. The setup allows for the application of well-controlled arbitrary load combinations at the four edges of the specimen (8 independent stress resultants or imposed displacements) by means of 100 hydraulic actuators (see Kaufmann et al. 2019 and LUSET).
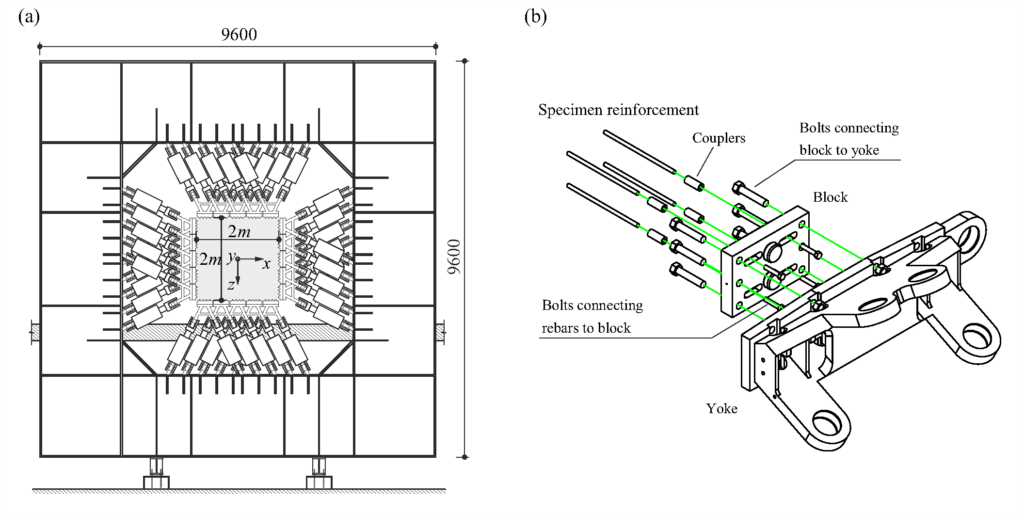
A total of 10 tests on orthogonally reinforced concrete shell elements were conducted. The shell elements, representing a part of a box-girder web, were subjected to combinations of homogeneous loading and imposed deformations, defined such that the boundary conditions imposed to the web by the overall part of the bridge structure were appropriately considered. The tested parameters, included the reinforcement ratio, the kinematic restraints and the loading sequence. In order to study the effect of the web’s stiffness degradation on the transverse bending moment distribution in a transverse section of the bridge, a so-called hybrid test was also conducted. In this test, a finite element model, which was continuously updated with the actual bending stiffness of the physical specimen, reflected the global behaviour of the transverse bridge section and accordingly determined the load to be applied.

What were the main experimental findings?
The results of the experimental campaign confirmed the basic assumption of the majority of existing rigid-ideally plastic models that the compression field responsible for the transfer of longitudinal shear will indeed shift laterally towards the flexural compression side of the web. The flexural compression acting on the shifted compression field together with the overall generalised reactions acting on the web, have a favourable effect on the web’s capacity against the combined loading. In all tests on members with very low stirrup reinforcement ratios, stirrup rupture was found to be the governing failure mode. This brittle failure mode, which occurred before a significant utilisation of the concrete had taken place, limits the applicability of existing models that do not explicity check the deformation capacity of the member. The experimental results therefore highlight the importance of comparing deformation demand to deformation capacity when assessing members with very low vertical reinforcement ratios.
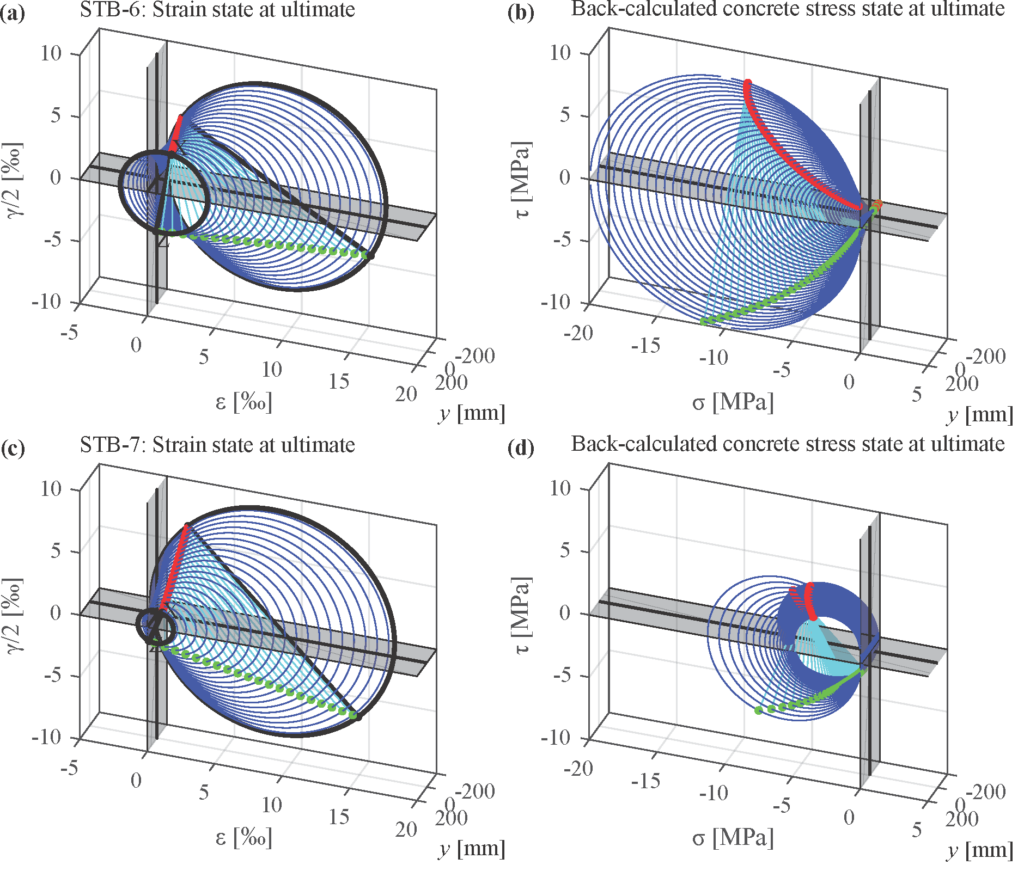
The conducted hybrid test, which accounted for system behaviour, confirmed that the redistribution of bending moments in a box-girder is favourable for the bearing capacity of the web against combined in-plane shear and transverse bending, provided that all adjoining structural elements (the deck and the bottom flange of the section) exhibit sufficient reserve capacity to accommodate the additional load.
Therefore, considering the system behaviour in the assessment of existing bridges can lead to a mitigation of required strengthening measures, or, if such measures are still deemed necessary, greatly simplify them by increasing the stiffness of the deck instead of performing more complicated strengthening measures.
How can existing methods be extended to include a check of the deformation capacity (while retaining the simplicity of application of those models)?
The extension of existing models to enable the check of the deformation capacity requires the consideration in their formulation of more complicated approaches for the modelling of the response of concrete and reinforcement (compression softening and tension stiffening in particular). This leads to their formulation becoming highly nonlinear therefore requiring complex iterative algorighms for their solution. In order to obtain a simplified procedure for the determination of the ultimate load, while at the same time respecting the limits of deformation capacity, a graphical approach was developed. This method, makes use of the experimental observations to reduce the problem to two kinematic unknowns and obtains an estimate of the ultimate transverse bending moment and the associated deformation by graphically determining the intersection of the solution space with the target values for the applied in-plane shear. In addition to providing the ultimate load, the method can also be utilised for the study of the system behaviour since it allows the calculation of simplified bilinear bending moment – curvature diagrams of the web at given levels of in-plane shear.
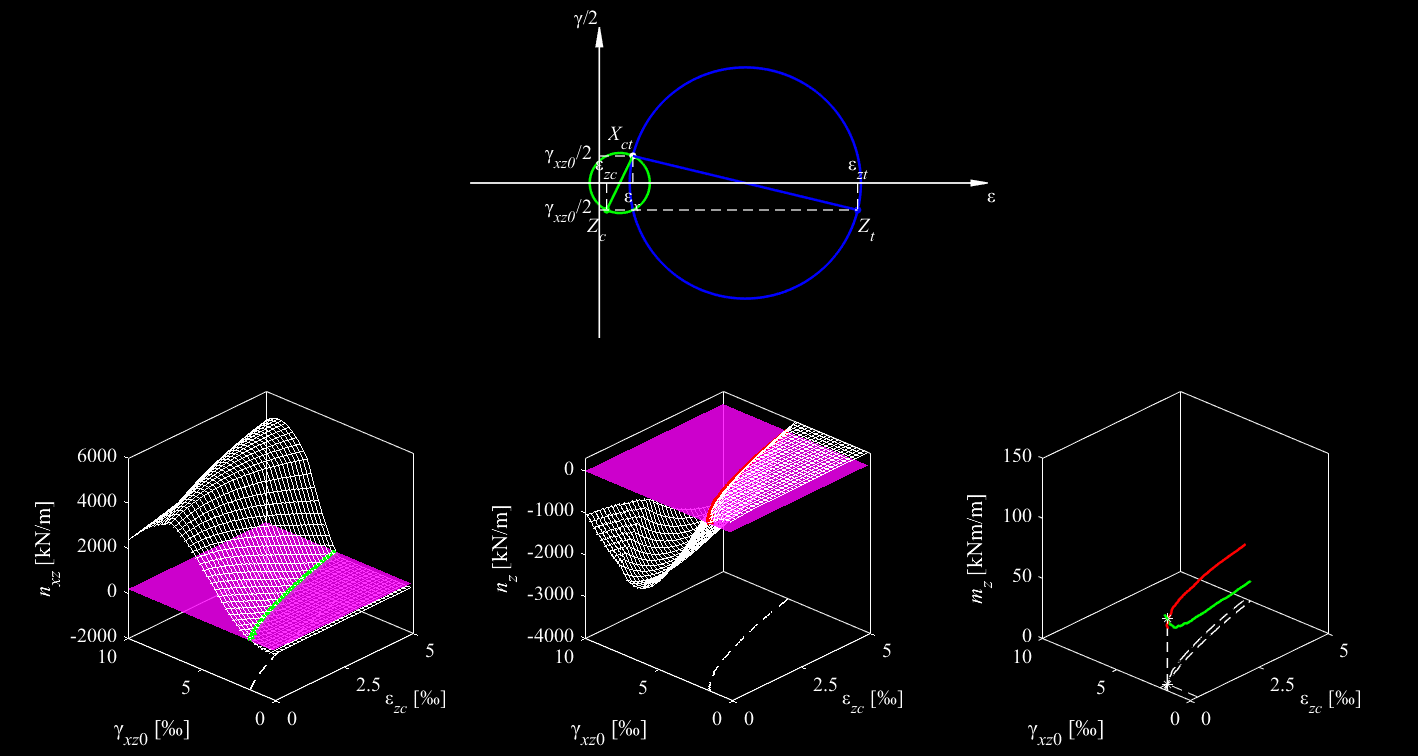
What is the main takeaway for practicing engineers?
In the assessment of existing structures, a great deal of effort is done towards maximising the utilisation of their existing load-carrying capacity. However, in the case of the combined loading under in-plane shear and transverse bending, a more realistic estimation of the transverse bending moment the web will actually be subjected to, is likely to have a greater impact in avoiding unnecessary strengthening measures compared to making maximum use of the existing load carrying capacity without considering a redistribution of load. Therefore, a more detailed study of the system behaviour accounting for the stiffness reduction in the web with increasing load is recommended.