Link zur deutschen Version: Umweltfreundliche Betonbauten?
Concrete – Foundation of urban civilisation
Concrete was indispensable for industrialisation and the development of our urban civilisation and is by far the most widely used building material today: around 15…20 billion cubic meters of concrete are cast annually, i.e. more than two cubic meters per world inhabitant(1). Concrete is this widely used because it unites good mechanical properties(2) with high durability(3) and fire resistance, combined with worldwide availability at low cost. The latter two advantages are often overlooked but are probably the most critical factors for the widespread use of concrete.
(1) Exact figures are not available. The estimate is based on the annual worldwide production of 3.99 billion tons registered by Cembureau (key figures Cembureau), extrapolated according to the procedure of the Cement Sustainability Initiative assuming 300 kg of cement with 70% clinker content per cubic meter of concrete. According to FSKB statistics, 15 million cubic meters of ready-mix concrete are supplied annually in Switzerland, which corresponds to an annual concrete consumption of around 2.5 cubic meters per capita given the 75% share of ready-mix concrete.
(2) Concrete has high compressive strength but fails in a brittle manner when subjected to minor tensile stresses. The invention of reinforced concrete, in which a reinforcement – usually in the form of reinforcing steel – resists tensile forces, was therefore essential to the success of concrete structures. On the other hand, the concrete ensures that the reinforcement heats up only slowly in the event of a fire, thus providing a high level of fire resistance. Reinforcing steel – around 1.6 million tons are used in Switzerland every year – is today produced almost exclusively from scrap metal.
(3) Thanks to the hydraulic properties of the cement, concrete is durable and even hardens underwater. The service life of weathered unreinforced concrete is therefore almost unlimited, as demonstrated, for example, by the Pantheon in Rome. This also applies to structural concrete, as long as the reinforcement does not corrode. If corrosion is an issue depends primarily on exposure: While there are no concerns in dry interiors, structures exposed to moisture and chlorides may need to be repaired after a few decades. Compared with most other building materials, however, the durability of reinforced concrete is very high even under such exposure.
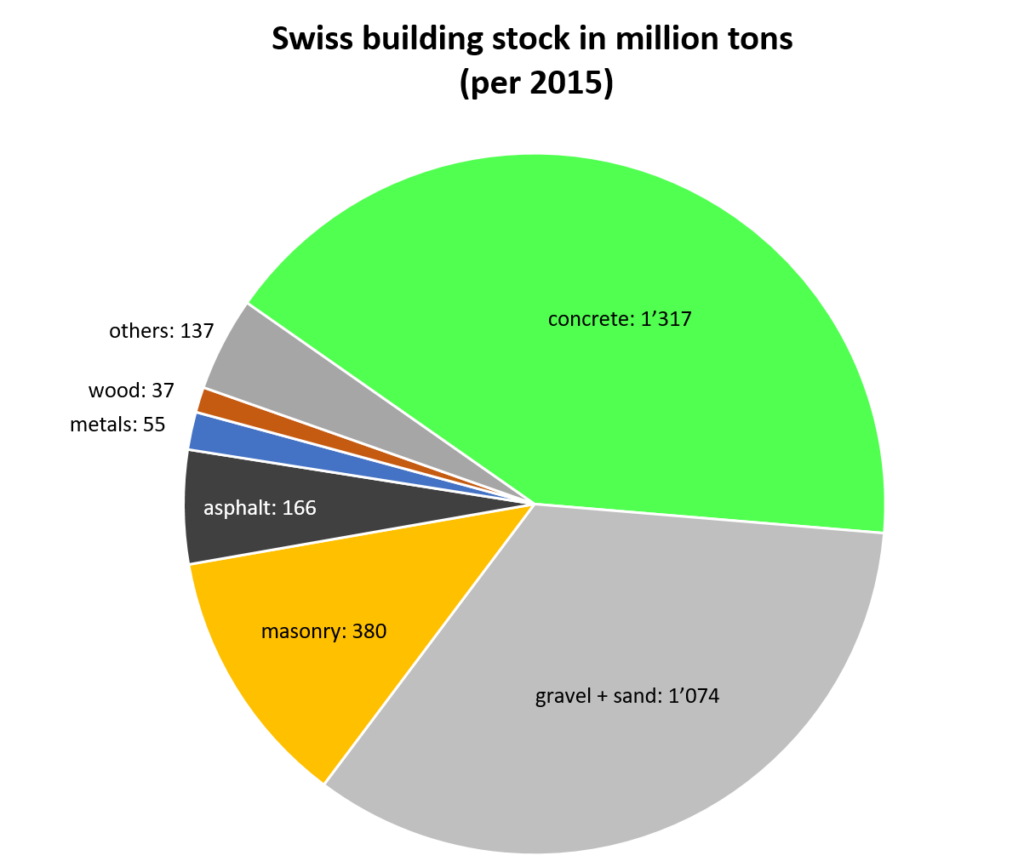
High resource consumption and greenhouse gas emissions
Obviously, the widespread use of concrete as a construction material is accompanied by high resource consumption, even though this can be somewhat improved by reusing demolished concrete as recycled aggregate for new concrete. In addition, the cement used as the binder in concrete contributes around 5…8% to global greenhouse emissions, depending on the calculation method(4). Therefore, in the light of climate change, calling for concrete to be replaced by other building materials, in particular wood, seems the obvious thing to do.
(4) The production of Portland cement (aka clinker) by calcining limestone and marl releases around 470 kg of CO2 per ton. Heating the raw materials to the temperature of approx. 1450°C required for calcination causes a further 100…500 kg CO2 per ton of clinker (depending on the fuel used). The high cement consumption of 3.99 billion tons per year (key figures Cembureau) – in Switzerland alone 4.21 million tons (key figures cemsuisse) – results in correspondingly high greenhouse gas emissions.
Replacing concrete: A viable solution?
However, the substitution of concrete is only partially possible and, at least on its own, not expedient. On the one hand, concrete is de facto without alternative for many applications: basements of buildings, retaining walls, dams or tunnels in other materialisation are far from being equivalent to concrete in load-bearing capacity, durability and costs. On the other hand, alternative building materials can only cover a small part of the quantities of building materials required worldwide(5). Moreover, they cause correspondingly high greenhouse gas emissions when built in large quantities, which in most cases exceed those of concrete(6).
(5) For example, annual logging worldwide today is about 2 billion cubic meters, of which about 500 million are processed into wood products (FAO Global Forest Products Facts and Figures 2018). This amount is equivalent to a few per cent of concrete consumption. Even if this amount were to be significantly increased (to the extent that this is feasible with sustainable forestry), only a small portion of concrete could be substituted.
(6) Here, solid timber is an exception, but many wood-based materials do cause high emissions as well. For example, plywood emits around six times more CO2 per cubic meter than concrete (KBOB Ökobilanzdaten im Baubereich (KBOB Ökobilanzdaten im Baubereich).
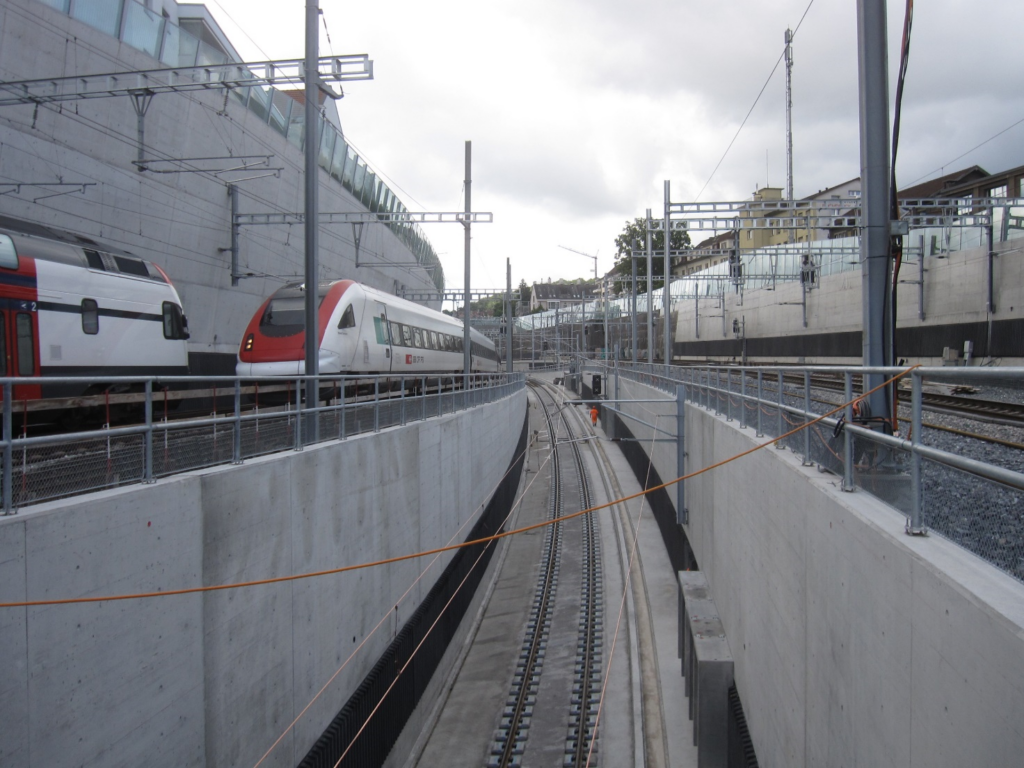
© dsp Ingenieure + Planer AG
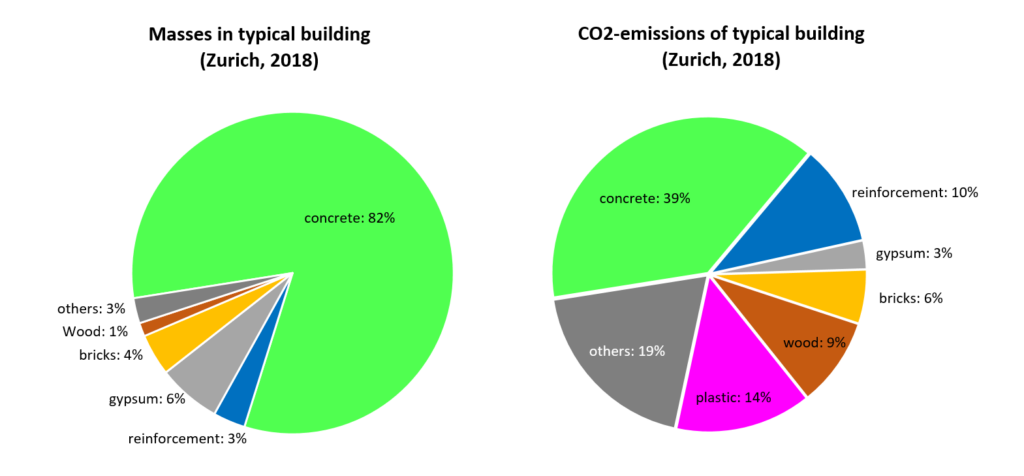
Therefore, if resource consumption and greenhouse gas emissions of the building industry are to be substantially reduced, concrete cannot simply be replaced. Instead, construction activity as a whole would have to be limited, combined with a transition to a circular economy. Such considerations are justified for industrialised countries: We already have a good infrastructure and can afford higher costs for its preservation, replacement or extension using alternative building materials – if necessary through incentive taxes to promote them. However, in developing and emerging countries, this is hardly realistic, and I find it presumptuous of industrialised countries to demand that these countries take such criteria into account when building their infrastructure or even ask for sacrifices. Bearing in mind that these countries today consume around 85% of the worldwide cement production (https://www.suedwind-magazin.at/zweischneidiges-schwert), replacing concrete is not really the solution.
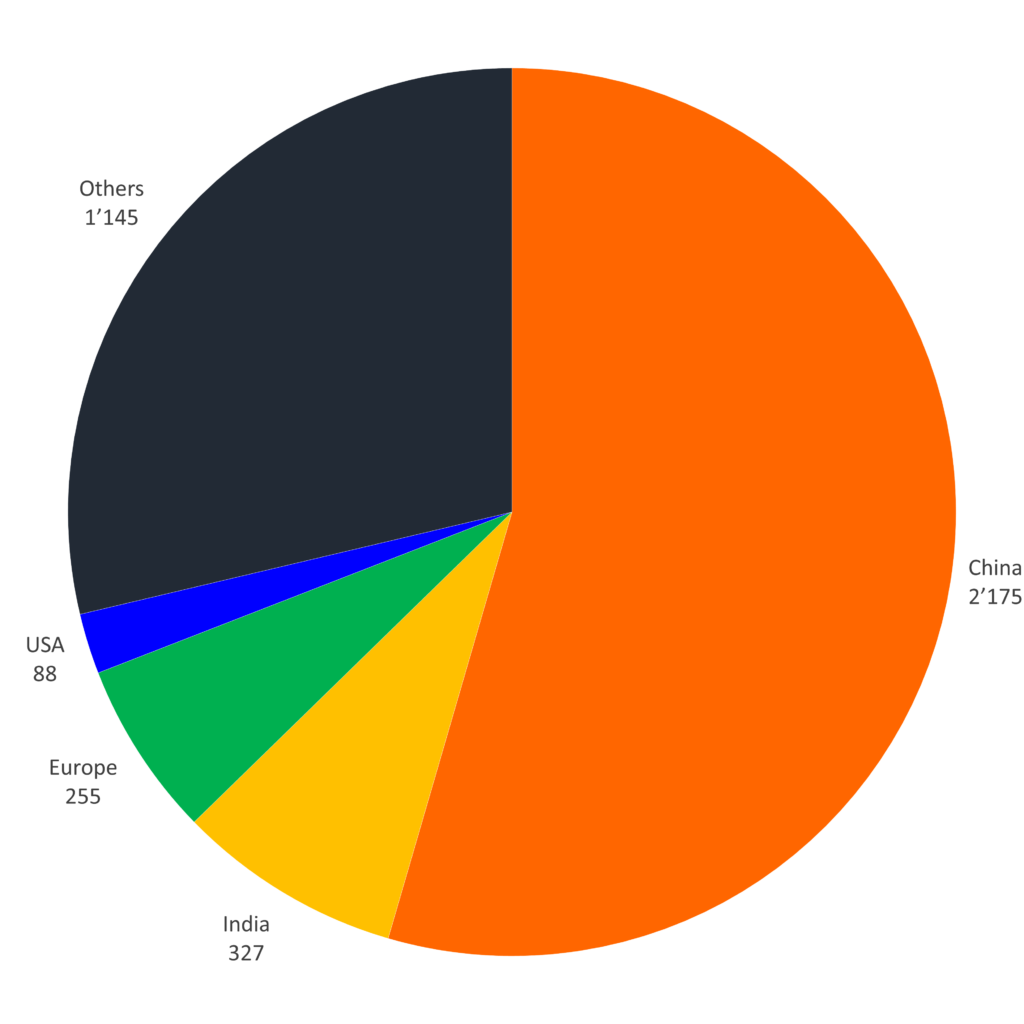
Environmental friendliness through structural efficiency
Rather, the goal must be to reduce resource consumption and emissions of concrete construction. To this end, Cembureau has developed the “5C” approach, which consists of the following five points:
- Clinker – reduction of fossil fuels used in clinker production
- Cement – reduction of the clinker content in cement
- Concrete – reduction of the cement content in concrete
- Construction – reduction of concrete consumption in buildings and structures
- Carbonation – increase carbonation
The first point is already largely implemented in Switzerland: Today’s Swiss cement industry uses more than two-thirds of alternative fuels for its energy needs. The clinker content in Swiss cement has also already been significantly reduced: The proportion of pure Portland cement, which was practically exclusively used until 1998, is now less than 7% (key figures cemsuisse). A further reduction of the clinker content in the cement is possible. However, the durability must not be impaired. For the latter reason, reducing the cement content in concrete is not straightforward either(7).
(7) Durability issues occur in concrete structures primarily due to reinforcement corrosion. The alkalinity of the concrete, which is ensured by the use of clinker, protects the reinforcement from corrosion. This must be taken into account when reducing the cement content and the clinker percentage in the cement for exposed concrete members.
Concrete buildings absorb 10-20% (depending on the calculation method) of the greenhouse gases emitted over their lifetime due to the carbonation of the concrete (CO2 uptake). This proportion can be increased, for example, through the targeted carbonation of crushed concrete granulate (https://www.aramis.admin.ch/Texte/?ProjectID=47360). An interesting alternative is to capture CO2 at an emission source and synthesise limestone from it, as the company Blue Planet is already doing. If such synthetic limestone is used as aggregate for concrete, concrete structures will absorb more CO2 in the future than is emitted by cement production. However, it is currently impossible to predict whether the process can be scaled up and operated economically.
Personally, I see the most significant potential in the reduction of concrete consumption, which can best be achieved by structural efficiency. Efficient structures transfer applied loads with low dead weight (and thus low resource consumption and emissions) in a material-appropriate way. This is where structural engineers can and must make their contribution to climate protection.
Due to low material prices and rising labour costs, structural efficiency has unfortunately all too often been neglected as a design goal in recent decades. Instead, simple structures that could be built quickly were favoured, even if they required considerably more material. In addition, minimum dimensions of concrete structures were continuously increased: In building construction to ensure sound insulation and to accommodate service ducts(8), and in civil engineering to ensure maximum durability(9). Here, the increasing pressure to reduce resource consumption and greenhouse gas emissions offers civil and structural engineers the opportunity to recover one of their very own areas of expertise and to restore the significance of structural efficiency to the status it deserves.
(8) An example of this are houses with controlled ventilation, as required to achieve the “Minergie” label. To be able to integrate the ventilation into the floor slabs, slab thicknesses are usually 26 cm. However, a much thinner slab would be sufficient from a structural point of view.
(9) The requirements for durability have been significantly increased due to experiences with corrosion damage to early concrete structures, which is undoubtedly justified: Premature replacement of a corroded structure is certainly not sustainable. However, the maximum durability requirements that many codes and clients today impose as a general rule on all structures should be questioned and differentiated, as they come at the expense of resource consumption and greenhouse gas emissions.
Efficient concrete structures
The most efficient concrete structures are undoubtedly anti-funicular arches and shells, which are lightweight and transfer applied loads primarily via compressive forces. However, this only applies to the final state. However, the conventional construction of such structures often requires very elaborate falsework and formwork, which significantly reduces their advantages
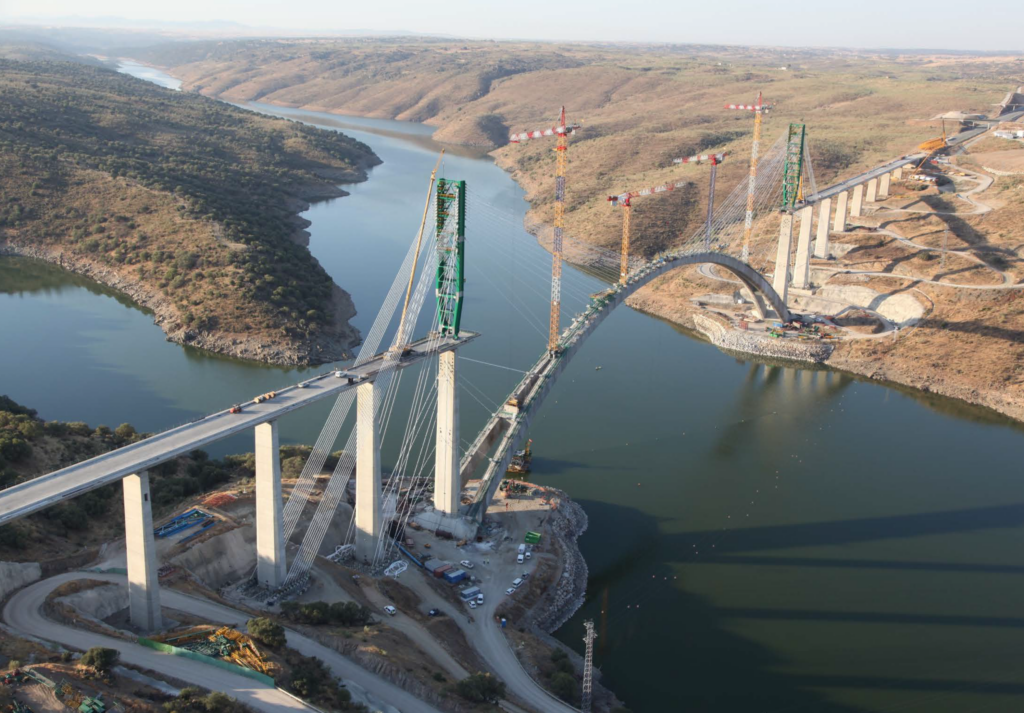
© Arenas & Asociados
Here, it is necessary to develop more efficient construction methods, which is, for example, a subject of current research in the NCCR dfab at ETH Zurich (https://dfab.ch/streams/lightweight-flexible-formwork). Such efforts are by no means new: for example, as early as 1892, J. Melan developed a system for constructing arches where a lightweight steel arch simultaneously serves as falsework for the concrete and its primary reinforcement. Today, arch bridges with spans of over 500m are being built in China using the “CSFT” (Concrete Filled Steel Tube) construction method based on the same principle (https://concrete.ethz.ch/brd/arch-bridges/).
By their curved nature, arches and shells are unsuitable for many applications, such as floor slabs, the most widespread application of reinforced concrete. However, even geometrically relatively simple structures can be structurally efficient if they are designed appropriately. There are some basic principles to be observed here that should be self-evident for structural engineers:
- Choose efficient structural systems: Continuous beams are much more efficient than simple beams
- Select efficient cross-sections: Profiled beams have much higher stiffness and resistance than rectangular cross-sections at the same weight
- Adapt girder height to load
- Consider construction process in design
For floors, ribbed, waffle and hollow core slabs are particularly suitable, typically enabling savings of more than 50% of the material compared with a solid slab. Here, as well, there are existing systems, such as standardised formwork for in-situ concrete coffered slabs and prefabricated hollow core floors. New possibilities are the subject of current research at the NCCR dfab at ETH Zürich.
Conclusion
Reducing resource consumption and greenhouse gases is imperative in view of climate change. However, replacing concrete with other building materials is only useful in a few cases, since the problem is not concrete per se but rather its widespread use: When other building materials are used in equally large quantities, they consume just as many resources and cause similarly large greenhouse gas emissions as concrete.
In fact, our buildings as a whole must become more environmentally friendly. By designing efficient structures, structural engineers can and must make a substantial contribution to climate protection. To ensure that such structures are also economic, the construction process must be part of the conceptual design process. Furthermore, we must develop construction methods enabling more complex structures to be built efficiently.
At our chair, we try to contribute by teaching the basics of efficient structural design and researching new fabrication methods.
Walter Kaufmann